Follow me:

Date posted: December 29th 2016
Book Title: Cosmos (continued)
Chapter 8: Light!
Alright enough of history, let’s look into space and nature once again. Let’s look at light. It seems nothing can go any faster than light. But it does have a limit. In vacuum, it travels at 1 billion km/h or 600 million mph—never any faster or any slower... However, this fixed speed in vacuum has its practicalities. In fact, it is thanks to that limit that we can calculate how far other stars and galaxies are. One of the methods to do this, is through the observation of events called “standard candles”. These are precise and repetitive explosions that always emit the same amount of light. And since we know their brightness we can calculate their distance relative to us by comparing the observed brightness (here on Earth) and their actual brightness (which, as I said is known).
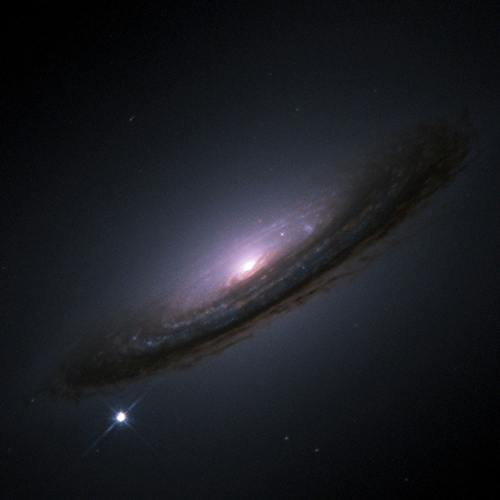
Novas and certain types of supernovas are known standard candles. The image above is a type-1a supernova1
Image under Creative Commons BY 3.0 license via Hubble Space Telescope
Moreover, we can calculate if those objects are moving towards us or away from us. This is done by identifying one of two phenomena, ‘blueshift’ or ‘redshift’. Blue shifting objects are moving towards us and red shifting objects are moving away from us. We have discovered that most of the objects at the limits of the observable universe are red shifting, that is moving away from us—and not at a constant speed, but at an accelerating rate! This means the universe is expanding faster and faster as time goes by.
Another curious thing about light in space, is that by the time it reaches us from a faraway object, the light that we detect here on Earth has been traveling for a long time. Meaning that if we observe a supernova explosion, it probably happened several years ago—but its light is just reaching us. The most bizarre examples of this ‘time dependent light travel’ are called quasars, these are some of the oldest objects in the observable universe. And chances are, such “quasars” are no longer in the state that classifies them as such. Billions of years have passed since light left them, and at this point they may have evolved to some other objects—perhaps galaxies or something we haven't even seen—and all we see are their ghosts. One hypothesis is that quasars are what young galaxies used to look like. The closest quasar is 2.3 billion light years from us! So when light left this quasar, life on Earth was unicellular and wouldn't become multicellular for another 1.7 billion years.
We may never reach a quasar, not only because they are extremely far away from us, but because they may no longer exist by the time we get there! However there is hope that one day humans (or whatever humans will be called a couple million years from now) will reach other stars within our galaxy. After all, as you will see in the next chapter, the Sun will die someday—and it would be a great idea to get the hell out of here before that happens.
Chapter 9: Out there...
Looking at limits is an excellent way to understand phenomena. Matter is one of these phenomena, and we have looked at its limits since before the common era. On the lower end of the spectrum, the extremely small, Democritus’ definition of the atom made us reflect on what the most basic unit of matter is. And as our civilization and technology advanced, we realized these unbelievably small particles (atoms) were made of even smaller particles. For example, Ernest Rutherford discovered the nucleus in 1909 by shooting radioactive particles at a thin gold film, and since then we have discovered dozens of particles that make up the nuclei.
On the other side of the spectrum, the extremely big, we have also made significant discoveries. Observing the stars has given us plenty of insight on how matter assembles and transforms. But the coolest thing about these two extremes, is that throughout history, parallel discoveries have been done on the upper limit and the lower limit (i.e. stars and atoms). Helium for example was detected in the Sun before it was found on Earth, hence its name from the greek god of the Sun, Helios.
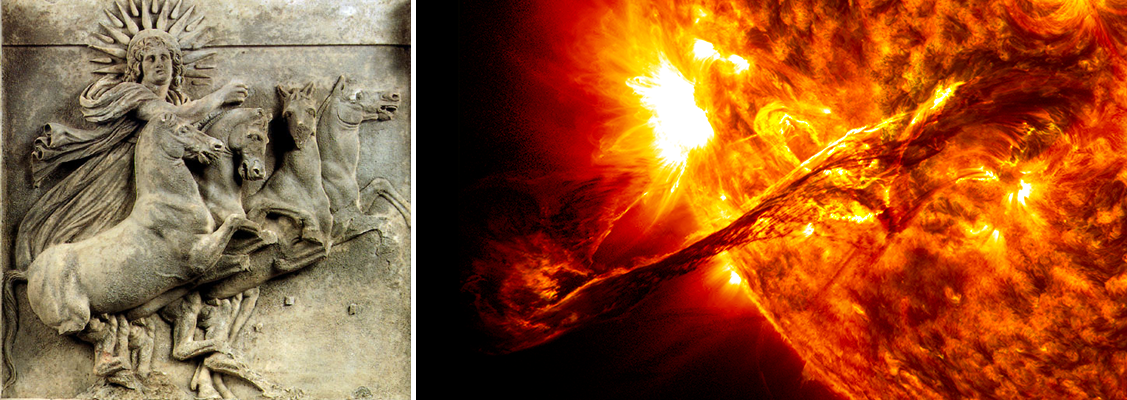
Helios the god of the Sun (left) and the Sun (right)
Images (left and right) under Public Domain via Wikimedia Commons and NASA respectively
Looking at the stars we have also understood their life cycles. Inside a star, gravity exerts such a high pressure that atoms are squeezed together (so close!) that they have no choice but to fuse together—and form a bigger atom. This process is called nuclear fusion, and it releases a tremendous amount of energy in the form of light and heat. Stars are fusion machines, and since their light is what sustains life, they are (potential) life breeding machines.
A relatively small star like the Sun fuses hydrogen into helium. However, its hydrogen supply is limited—and a couple billion years from now the Sun will start running out of fuel. When that happens, a sequence of events will unfold—and the Sun will enter into its long and agonizing death. The first one of these events will be the migration of the fusion reaction towards the surface. When it reaches the surface it will stop momentarily, but gravity will pull the remains back together (mostly helium at this point), collapsing again and sparking yet another fusion reaction—but this time helium fusion (a bigger atom than hydrogen).
Some larger stars can do this fusion “step‑up” more than once as they die, but the Sun doesn't have enough mass and it will only step up once—to helium. The helium fusion on the dying Sun may last for a few millennia, but this whole time the Sun will have a strong temperature gradient between the hot helium fusion in the core, and the slightly cooler red surface. This gradient will cause it to expand, most likely reaching the orbits of Mercury, Venus, and perhaps the Earth. At this point stars are called ‘red giants’ and they are very unstable.
In this red giant state, the Sun will collapse gradually through violent contractions—during which millions of tons of stellar dust will be shed into space. Each contraction will result in a “smaller giant” and a larger dust cloud (sometimes called a planetary nebula). When enough stellar dust has been shed, the red giant will shrink into something more like a ‘red dwarf’—with a huge planetary nebula around it.
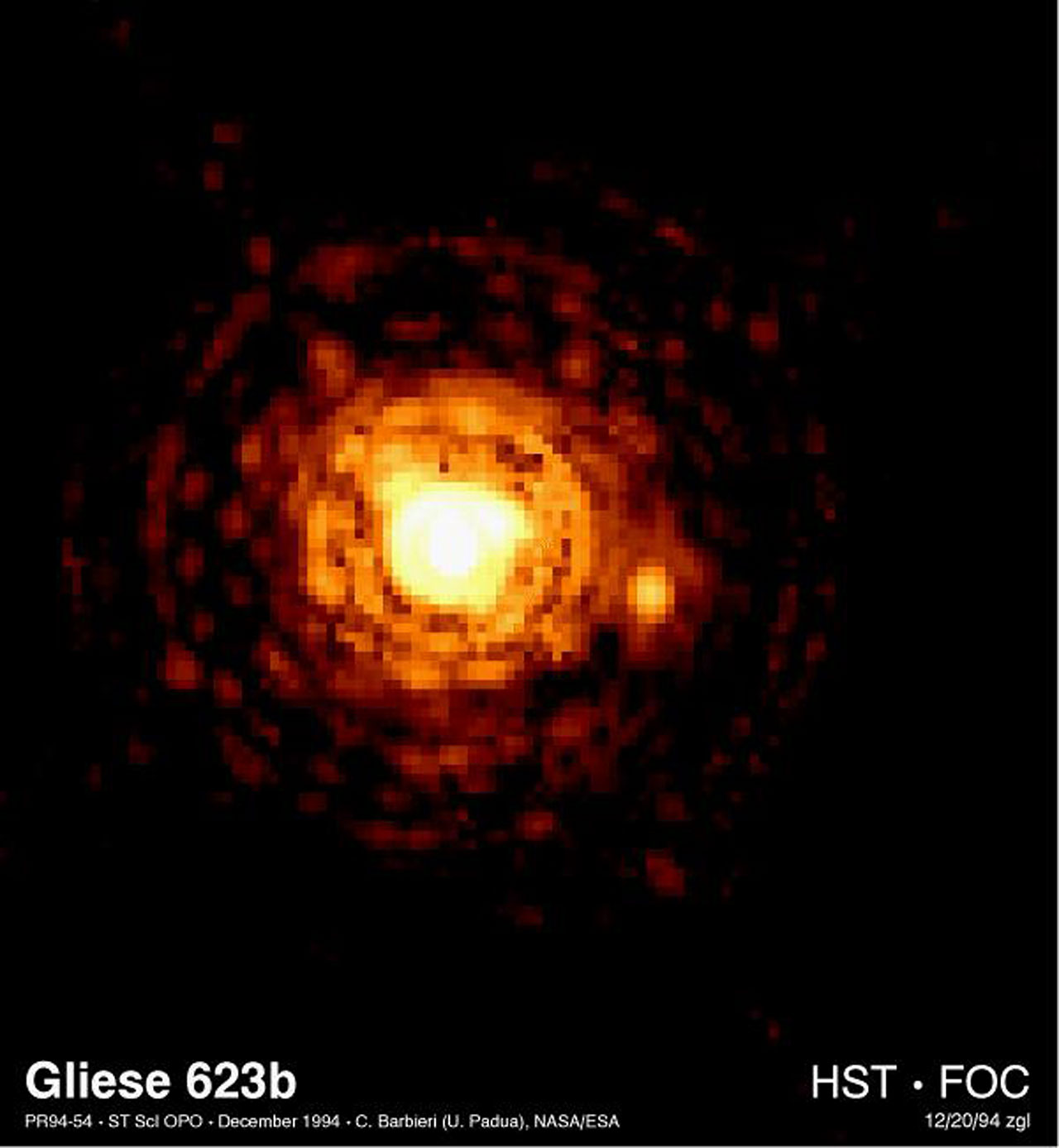
One of the smallest stars in our Milky Way Galaxy can be found in a binary system of two red dwarfs called Gliese 623b. We’ll talk about binary systems later but for now, just notice there are two here. The one in the center which is pretty obvious, but there’s a tiny little fireball to the right of it. That tiny star is ten times less massive than the Sun and 60,000 times fainter. You can also appreciate the nebula around them
Image under Creative Commons BY 3.0 license via Hubble Space Telescope
Towards the end of this ‘red giant’ phase, there will be a point where there is not enough mass for a sustained fusion reaction—and it will simply stop. The red‑dwarf Sun will turn white and will slowly cool down for billions of years. At this point it will be called a “white dwarf”, characterized by its color and the stellar dust bubble surrounding it. Finally, once cold enough (and this takes a long long time) it will become a dead and sad “black dwarf”.
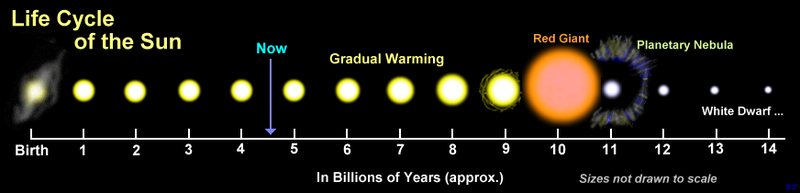
I found this cool scale. It’s missing some detail but shows (in a nutshell) the life cycle of the Sun. If you are wondering at what point the Earth was formed, well almost at the same time as the Sun was formed
Image under Public Domain via Wikimedia Commons
Now, I mentioned binary systems in one of the pictures above. A binary system in astronomy means two things orbiting each other. It could be two stars, two black holes, two asteroids—pretty much anything, as long as they are similar in size and gravitationally stable. And speaking of dying stars, binary stars have very different fate than our lonely Sun.
You see, it is very likely that ONE of those two stars will become a white dwarf before the other one does. And a white dwarf next to a red giant has a very peculiar death. Because when the red giant begins pulsating (like the our dying Sun above), its stellar dust will be attracted by the white dwarf’s gravity. This gas exchange triggers a series of explosions called Novae.
Carl did not explicitly say what happens later but I assume (if the mass is balanced) they will eventually both turn into white dwarfs and cool slowly into black dwarfs. Although I found online someone saying that “when white dwarf stars reach the Chandrasekhar limit of 1.4 solar masses, they explode, completely annihilate, nothing is left behind”2.
Ok, moving on to bigger stars. Single stars that are much bigger than the Sun have a different death as well. When their fusion stops (often silicon fusion, which is the third ‘step‑up’), these massive stars contract until there is barely any space between the atomic nuclei. And they explode vividly in one of the most magnificent events in the universe, known as a supernova. A supernova is also a fusion reaction, but it happens in a matter of seconds. It releases a bunch of heavy elements into space, and for a few moments, this single star becomes brighter than the entire galaxy where it sits.
A huge nebula is left behind, but also a collection of neutrons right at the center. These are called neutron stars. If you think about it, this supernova event is quite similar to the white dwarf case, but the nebula is much bigger and the star in the center is much smaller. Neutron stars are often rotating very rapidly and producing some of the highest magnetic fields we’ve ever detected. However they don't emit much visible light. They are as big as a small city and each teaspoon of it, weighs as much as entire mountain ranges on Earth.
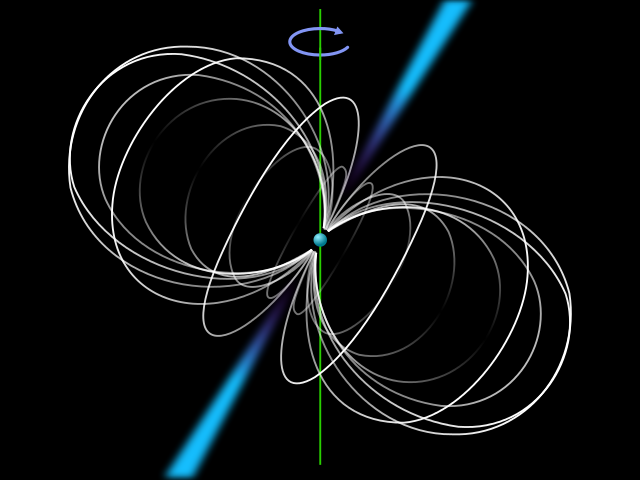
When a neutron star rotates very rapidly it often emits pulses of radiation and when they do they are called pulsars. This is a sketch of a pulsar. The sphere in the middle represents the neutron star, the curves indicate the magnetic field lines, the protruding cones represent the radiation emission beams, and the green line represents the axis on which the star rotates
Image by Mysid under Creative Commons BY-SA 3.0 license via Wikimedia Commons
The nebula from a supernova contains heavy elements produced during the explosion, and we believe this is how the iron, the gold, the silver, and pretty much every element found on Earth was made—in a matter of seconds. Certain places in such nebulae may still have enough mass to bind together by gravity and form smaller stars and planets. So in a way, the death of a big star can give birth to smaller, more stable stars with planetary systems like ours. As Carl used to say “we are made of star stuff”.
Finally, stars more massive than those who explode in a supernova, have an even more astonishing and mysterious destiny. Instead of leaving a neutron star behind, they leave a back hole behind. Objects so dense that their gravity doesn't even let light out. These explosions are often called hypernovae. They also explode in a very strange way, check out this animation from NASA
Click on the Next button to continue reading